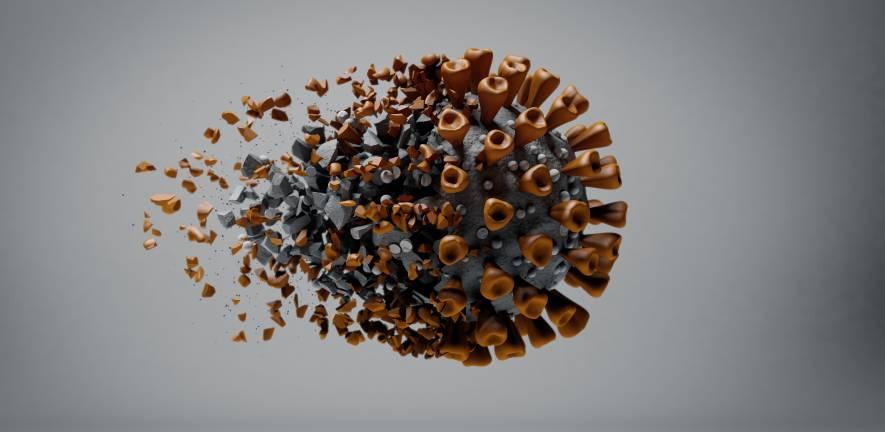
Cambridge scientists have used synthetic biology to create artificial enzymes programmed to target the genetic code of SARS-CoV-2 and destroy the virus, an approach that could be used to develop a new generation of antiviral drugs.
Cambridge scientists have used synthetic biology to create artificial enzymes programmed to target the genetic code of SARS-CoV-2 and destroy the virus, an approach that could be used to develop a new generation of antiviral drugs.
XNAzymes are molecular scissors which recognise a particular sequence in the RNA, then chop it up
Alex Taylor
Enzymes are naturally occurring biological catalysts, which enable the chemical transformations required for our bodies to function – from translating the genetic code into proteins, right through to digesting food. Although most enzymes are proteins, some of these crucial reactions are catalysed by RNA, a chemical cousin of DNA, which can fold into enzymes known as ribozymes. Some classes of ribozyme are able to target specific sequences in other RNA molecules and cut them precisely.
In 2014, Dr Alex Taylor and colleagues discovered that artificial genetic material known as XNA – in other words, synthetic chemical alternatives to RNA and DNA not found in nature – could be used to create the world’s first fully-artificial enzymes, which Taylor named XNAzymes.
At the beginning, XNAzymes were inefficient, requiring unrealistic laboratory conditions to function. Earlier this year, however, his lab reported a new generation of XNAzymes, engineered to be much more stable and efficient under conditions inside cells. These artificial enzymes can cut long, complex RNA molecules and are so precise that if the target sequence differs by just a single nucleotide (the basic structural unit of RNA), they will recognise not to cut it. This means they can be programmed to attack mutated RNAs involved in cancer or other diseases, leaving normal RNA molecules well alone.
Now, in research published today in Nature Communications, Taylor and his team at the Cambridge Institute of Therapeutic Immunology & Infectious Disease (CITIID), University of Cambridge, report how they have used this technology to successfully ‘kill’ live SARS-CoV-2 virus.
Taylor, a Sir Henry Dale Fellow and Affiliated Researcher at St John’s College, Cambridge, said: “Put simply, XNAzymes are molecular scissors which recognise a particular sequence in the RNA, then chop it up. As soon as scientists published the RNA sequence of SARS-CoV-2, we started scanning through looking for sequences for our XNAzymes to attack.”
While these artificial enzymes can be programmed to recognise specific RNA sequences, the catalytic core of the XNAzyme – the machinery that operates the ‘scissors’ – does not change. This means that creating new XNAzymes can be done in far less time than it normally takes to develop antiviral drugs.
As Taylor explained: “It’s like having a pair of scissors where the overall design remains the same, but you can change the blades or handles depending on the material you want to cut. The power of this approach is that, even working by myself in the lab at the start of the pandemic, I was able to generate and screen a handful of these XNAzymes in a matter of days.”
Taylor then teamed up with Dr Nicholas Matheson to show that his XNAzymes were active against live SARS-CoV-2 virus, taking advantage of CITIID’s state-of-the-art Containment Level 3 Laboratory – the largest academic facility for studying high risk biological agents like SARS-CoV-2 in the country.
“It's really encouraging that for the first time – and this has been a big goal of the field – we actually have them working as enzymes inside cells, and inhibiting replication of live virus,” said Dr Pehuén Pereyra Gerber, who performed the experiments on SARS-CoV-2 in Matheson’s lab.
“What we’ve shown is proof of principle, and it’s still early days,” added Matheson, “It’s worth remembering, however, that the amazingly successful Pfizer and Moderna COVID-19 vaccines are themselves based on synthetic RNA molecules – so it’s a really exciting and rapidly developing field, with enormous potential.”
Taylor checked the target viral sequences against databases of human RNAs to ensure they were not present in our own RNA. Because the XNAzymes are highly specific, this should in theory prevent some of the ‘off-target’ side-effects that similar, less accurate molecular therapeutics may cause, such as liver toxicity.
SARS-CoV-2 has the ability to evolve and change its genetic code, leading to new variants against which vaccines are less effective. To get around this problem, Taylor not only targeted regions of the viral RNA that mutate less frequently, but he also designed three of the XNAzymes to self-assemble into a ‘nanostructure’ that cuts different parts of the virus genome.
“We’re targeting multiple sequences, so for the virus to evade the therapy it would have to mutate at several sites at once,” he said. “In principle, you could combine lots of these XNAzymes together into a cocktail. But even if a new variant does appear that is capable of getting round this, because we already have the catalytic core, we can rapidly make new enzymes to keep ahead of it.”
XNAzymes could potentially be administered as drugs to protect people exposed to COVID-19, to prevent the virus taking hold, or to treat patients with infection, helping rid the body of the virus. This sort of approach might be particularly important for patients who, because of a weakened immune system, struggle to clear the virus on their own.
The next step for Taylor and his team is to make XNAzymes that are even more specific and robust – “bulletproof,” he says – allowing them to remain in the body for longer, and work as even more effective catalysts, in smaller doses.
The research was funded by the Wellcome Trust, the Royal Society, the Medical Research Council, NHS Blood and Transplant, and Addenbrooke’s Charitable Trust.
Reference
Pereyra Gerber, P, Donde, MJ, Matheson, NJ and Taylor, AI. XNAzymes targeting the SARS-CoV-2 genome inhibit viral infection. Nature Communications (2022). DOI: 10.1038/s41467-022-34339-w
The text in this work is licensed under a Creative Commons Attribution 4.0 International License. Images, including our videos, are Copyright ©University of Cambridge and licensors/contributors as identified. All rights reserved. We make our image and video content available in a number of ways – as here, on our main website under its Terms and conditions, and on a range of channels including social media that permit your use and sharing of our content under their respective Terms.