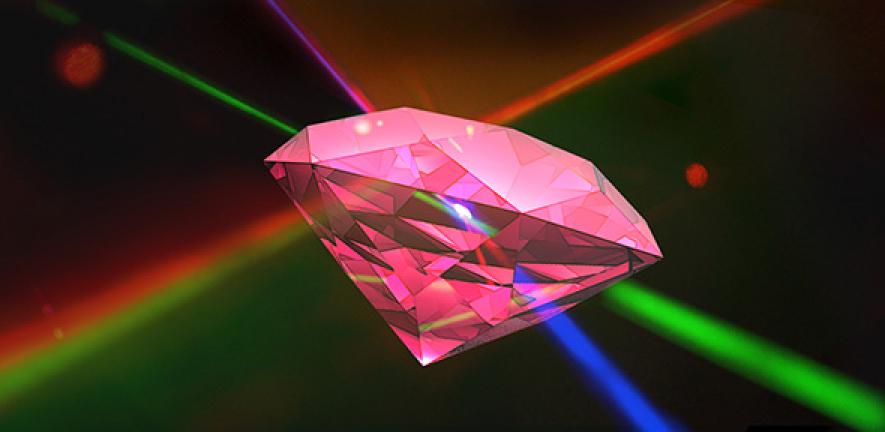
Defects in microscopic diamonds caused by the presence of silicon could provide researchers with a potent basis for developing new technologies, including nanoscale sensing devices.
Defects in microscopic diamonds caused by the presence of silicon could provide researchers with a potent basis for developing new technologies, including nanoscale sensing devices.
This research could eventually enable us to see what goes on inside cells at an atomic level
Mete Atature
Scientists have successfully tested a new way of using miniscule fragments of diamond to transmit information, a method which could eventually lead to the development of new computing and sensing technologies.
The research, reported today (Tuesday, 18 February, 2014) in the journal Nature Communications involved exploiting atomic defects which appear in the crystal structure of a diamond, known to physicists as “vacancy centres”.
These are literally vacancies – gaps in the lattice of carbon atoms which make up diamond at its most fundamental level. They usually occur around an impurity, where instead of a carbon atom, some other element has naturally found its way into the structure.
For scientists, vacancy centres offer huge promise because they trap electrons which could then be manipulated to transmit information in a radically new way. Eventually, the technique could enable the development of quantum networks and quantum computing; a means of moving data far more efficiently and quickly than computers do today.
Yet the perfect vacancy centre is hard to come by, because it needs to possess a combination of very precise characteristics which are rarely found together. The new study marks a breakthrough because researchers managed to access the state of the electrons around a vacancy centre based on silicon – something which had not been done before – and discovered that it has some of the crucial qualities that they have been looking for.
The work was carried out by a team of researchers from the University of Cambridge, UK, and Saarland University, in Germany.
Dr Mete Atatüre, a Fellow of St John’s College and researcher at the Cavendish Laboratory, who co-led the project with Professor Christoph Becher, said: “This is something that our community has been working on for years, but really we are still just at the beginning. The main advantage of the silicon vacancy centre is that its internal state is transferred to better quality photons, with a cleaner spectrum, when compared with other sources.”
Diamonds are naturally full of impurities, of which hundreds of different types exist. Their appearance within the carbon structure change the diamond’s properties and it is their interaction with light that gives a diamond its colour. “Ironically, what makes diamond precious is the fact that it’s impure,” Atatüre said. “Synthetic diamond, grown in lab conditions so that is completely pure, looks like a piece of glass; there’s nothing attractive about it visually.”
By isolating the impurity in a microscopic fragment of diamond, researchers can hold the electrons trapped in the vacancy in a single place, and then manipulate them.
They are trying to change a property of the electrons known as “spin”, a reference to their momentum, which can either point up or down. The idea behind quantum computing is to control the spin of electrons so that the up and down spins correspond to the 0s and 1s that represent information in binary code. The information is then transmitted with photons, so that it can be registered somewhere else.
Potentially, the technique would enable the development of super-fast computers, and effectively transmitting information from the electrons trapped in the vacancy centre is therefore the holy grail of this type of research.
Doing it successfully, however, depends on the type of vacancy centre being used. First the spin properties must be good enough to make them usable. Then the imprint of that spin, which decays in a matter of microseconds, needs to last long enough for it to be transmitted – a factor known as “coherence”. Finally, the photons – the light particles which actually convey the information – need to be high quality with clean spectral properties.
“What we’re looking for is a vacancy centre that behaves well in terms of its spin, has long coherence, and a photon that is bright enough and on a sufficiently narrow bandwidth to be useful,” Atatüre explained.
Most current research of this type uses nitrogen as the impurity in the diamond. Nitrogen vacancy centres are very abundant, and their spin properties are extremely good. Unfortunately, their optical properties are less spectacular – the broad bandwidth of their optical signature means that the imprint the electron spin leaves on the photon is lost in the vast majority of cases.
In the new study, the two research teams opted to use silicon instead, because it looked to have more promising optical qualities. This was first added to lab-grown diamonds. Next, the team used a combination of magnetic field and polarised light to access the spin of the electrons trapped in the silicon vacancy centre.
As well as being able to observe quantum states within the diamond, they also found that the fluorescence was brighter than many other systems and had a narrower bandwidth. This enabled them to correlate the spin properties of the trapped electron with the properties of the emitted photon. As a result, the Saarland and Cambridge research teams were then able to carry out further research assessing fully the electronic structure of the defect itself (published last month in Physical Review Letters).
Future research will focus on reversing the process, and directing laser light at the diamond itself in an effort to manipulate the spin into arbitrary quantum states, in an effort to maximise the potential of silicon vacancy centres.
Although the driving potential of such work for many researchers remains quantum technologies, the optical qualities of spin-based devices could also be exploited to create microscopically-small nano-scale sensors. These could not only be used to monitor and fix nanoelectronic devices, but also to unlock the secrets of biological cells, including our own.
“If we are less blinkered than just using this research to develop quantum computing, the potential to develop new types of sensors is huge,” Atatüre added. “In biology, we still do not understand the complexity of much of what is happening inside a single cell. This research could enable us eventually to see what goes on inside cells at an atomic level.”
For more information about this story, please contact Tom Kirk, Tel: +44 (0)1223 768377, tdk25@cam.ac.uk
This work is licensed under a Creative Commons Licence. If you use this content on your site please link back to this page.