Sight and sound
How photoacoustics could transform cancer detection and monitoring
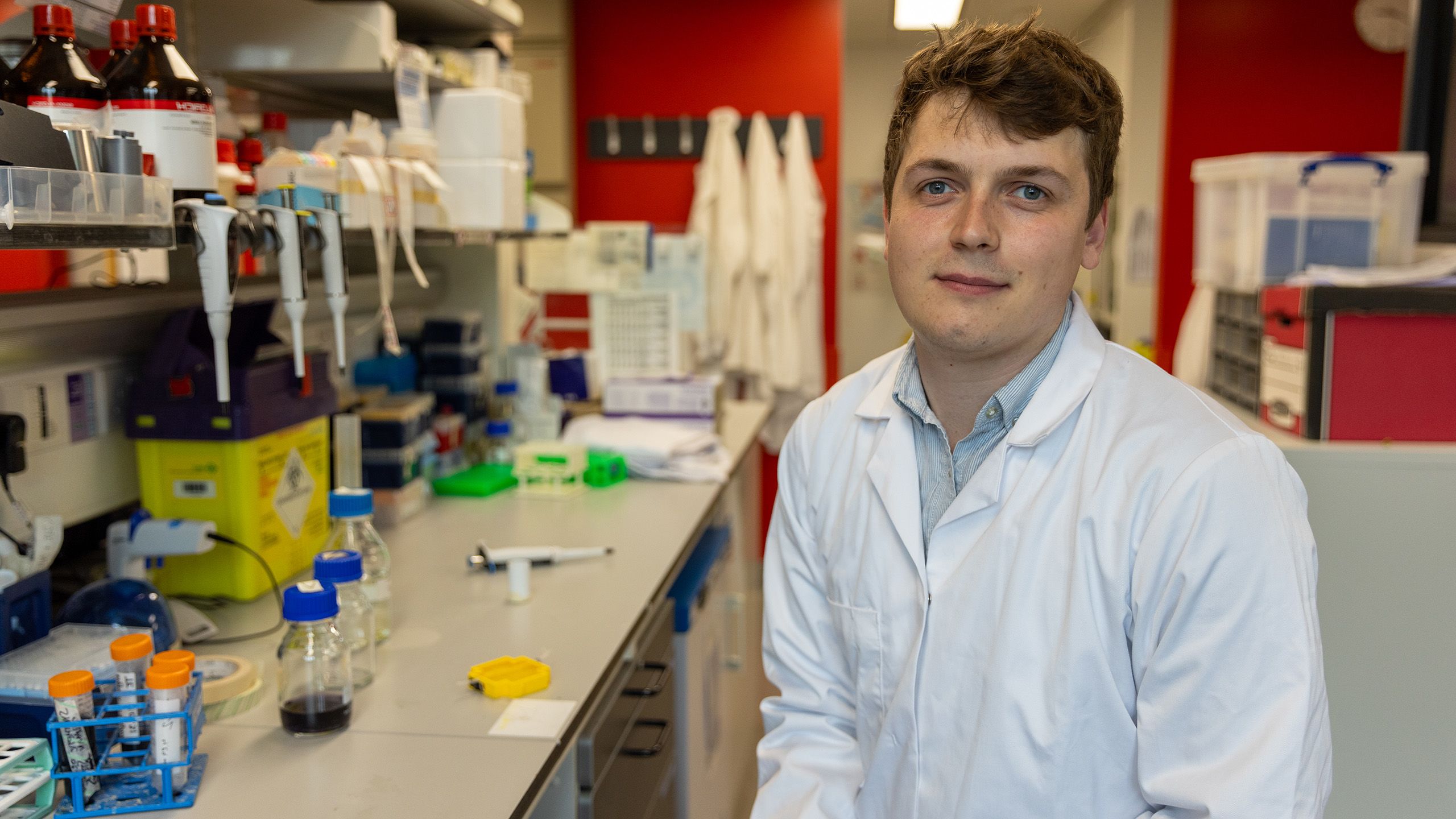
Combining light and ultrasound could offer a safer, more portable method for detecting and monitoring cancer, say Cambridge physicists – but they say we have to make sure devices work for people of different skin tones.
The University of Cambridge’s Cavendish Laboratory – celebrating its 150th anniversary and soon moving to its new home, the Ray Dolby Centre – is one of the most legendary physics laboratories in the world. It’s where the electron, the neutron and the structure of DNA were discovered. To date, 30 Cavendish researchers have been awarded the Nobel Prize.
Although primarily associated with physics, today, researchers at the Cavendish are also helping to unravel the mysteries of cancer. This includes Professor Sarah Bohndiek, who is jointly based at the Cavendish and the CRUK Cambridge Institute.
Bohndiek’s team specialises in the use of light to uncover hidden properties of disease, especially cancer. They are developing devices that go beyond the visible spectrum, using different wavelengths of light to enable earlier detection.
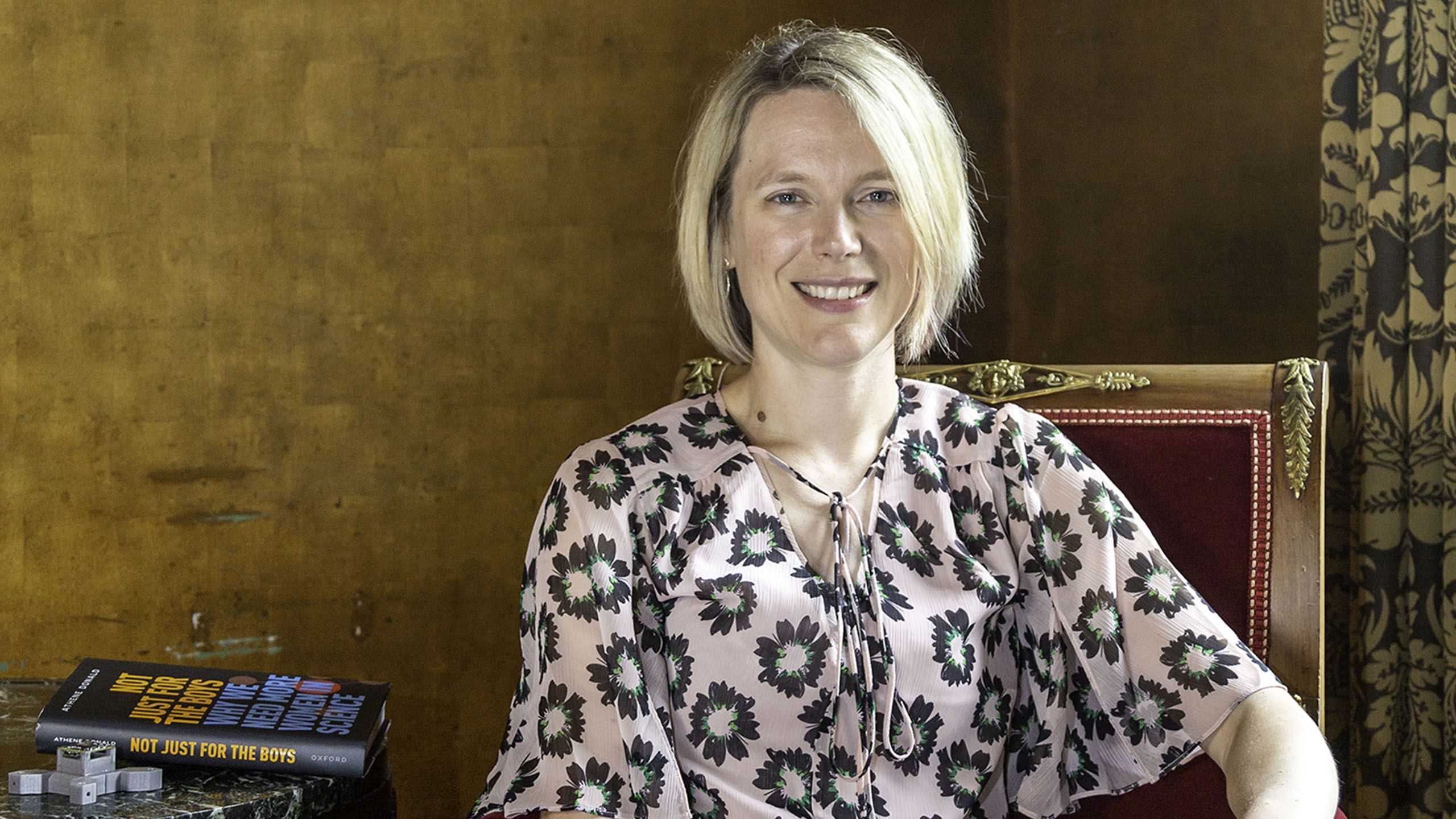
Sarah Bohndiek
Sarah Bohndiek
One of their key projects involves photoacoustic imaging, a technology combining light and ultrasound. Normal ultrasound scanners send sound into the body and measure the echo. Photoacoustic imaging systems, however, measure the ultrasound generated when molecules absorb light and heat up.
Photoacoustic images show how molecules in the body are changing and are particularly useful for measuring oxygen levels in the blood. “This is a useful feature for looking at cancer,” said Thomas Else, a research associate in Bohndiek’s team. “Cancers tend to have lower oxygen levels than healthy tissue because they grow so quickly.”
Most widely-used methods for detecting and monitoring cancer – such as MRI and X-ray mammography machines – are bulky and expensive pieces of equipment, and in the case of mammography uses ionising radiation and applies painful compression.
“The lack of ionising radiation makes photoacoustic imaging safe and suitable for long-term monitoring, and it can produce high-contrast images at the bedside, like an ultrasound,” said Else. “Photoacoustic systems are also lower cost and more portable than MRI machines, which makes it a valuable cancer monitoring technique in a wider range of medical settings.”
Photoacoustic imaging is already CE-marked in Europe and FDA-approved in the United States for breast cancer detection which could be a cost-effective alternative to traditional mammograms.
However, one of the challenges with the application of photoacoustic imaging, like several light-based medical technologies, is that it does not perform as well for people with darker skin tones. Melanin, the main pigment that affects skin tone, absorbs light, meaning less light can get through the skin to make a measurement.
Typically, photoacoustic imaging uses light from the red end of the visible spectrum to the infrared – at wavelengths around 700 to 900 nanometres. In this range, haemoglobin in the blood is the main source of contrast. But in darker-skinned patients, melanin can overwhelm the haemoglobin signal.
“Melanin in the skin blocks some light and prevents it from getting deep into the tissue and to the cancer or the organ we want to image,” said Else. “We wanted to look at the physics behind the underperformance of light-based imaging technologies for people of colour, which could help us address it.”
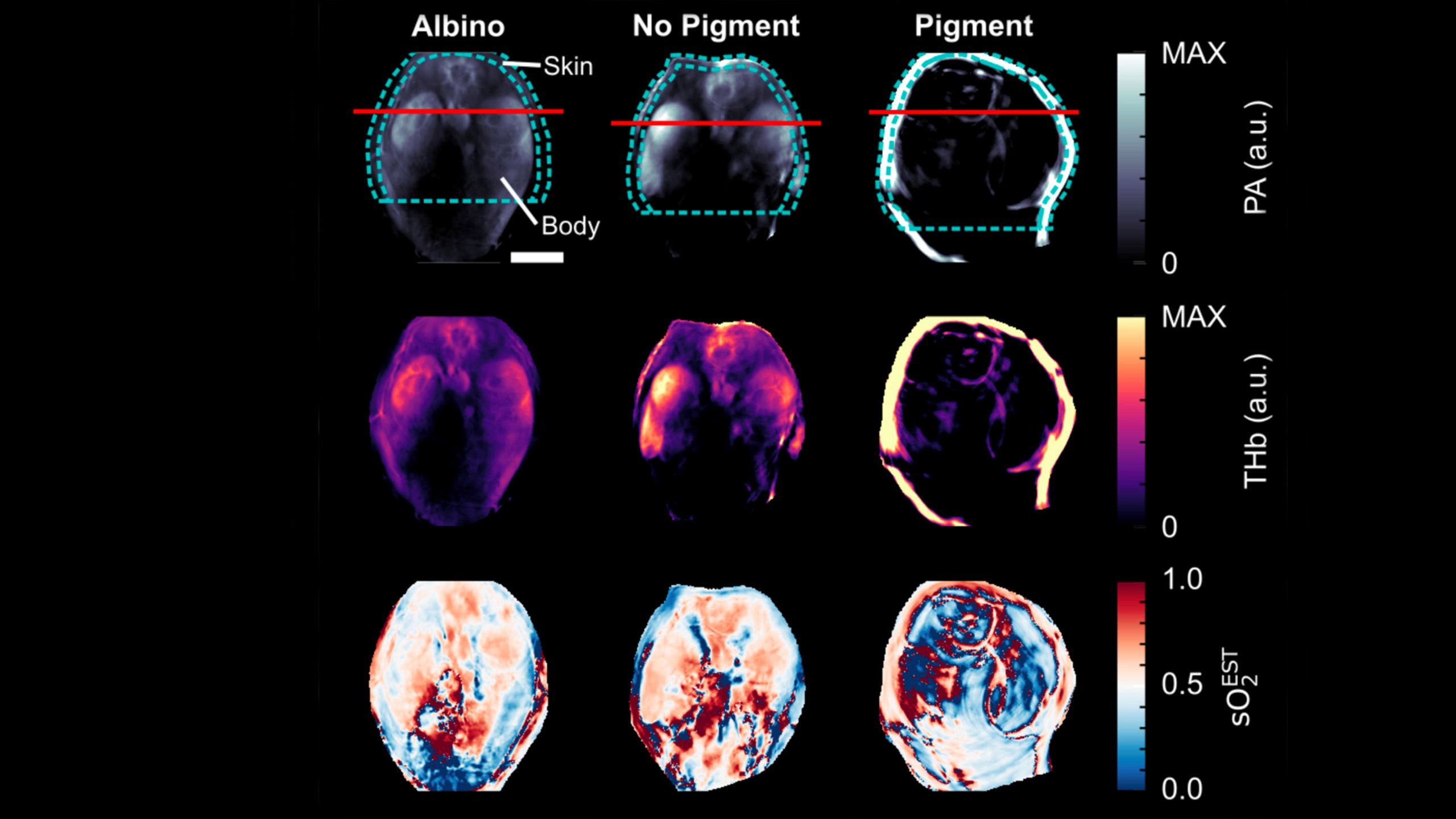
Photoacoustic images of mouse spleen and kidneys in albino and pigmented mice
Photoacoustic images of mouse spleen and kidneys in albino and pigmented mice
In a study recently published in the Journal of Biomedical Optics, Else and his colleagues used a computer model of skin with varying levels of pigmentation to simulate photoacoustic images. They validated the results in mice with different pigmentation patterns and in gel models, called phantoms, that mimic different skin tones.
“We found two main effects: one was if you try to measure blood oxygenation – which is important in cancer – in people with dark skin, you get incorrect measurements,” said Else. “Blood oxygenation in Black patients is overestimated compared to white patients. You also get lower quality images, with noise and distortions in the image, that could lead a clinician to misinterpret the scan, potentially making a tumour look more aggressive than it really is.”
To address these discrepancies, the researchers are conducting further studies with volunteers at Addenbrooke’s Hospital in collaboration with the Alliance for Cancer Early Detection to refine the technology and ensure its efficacy across diverse skin tones.
Bohndiek’s team is also exploring the use of photoacoustics for tailoring radiotherapy. By using photoacoustics to understand how cancers respond to treatment, clinicians might be able to customise radiation doses more effectively.
The researchers are also exploring other light-based technologies, like pulse oximeters and smartwatches, which have shown biases in blood oxygenation readings for Black patients. They aim to develop wearable devices that provide accurate measurements regardless of skin tone.
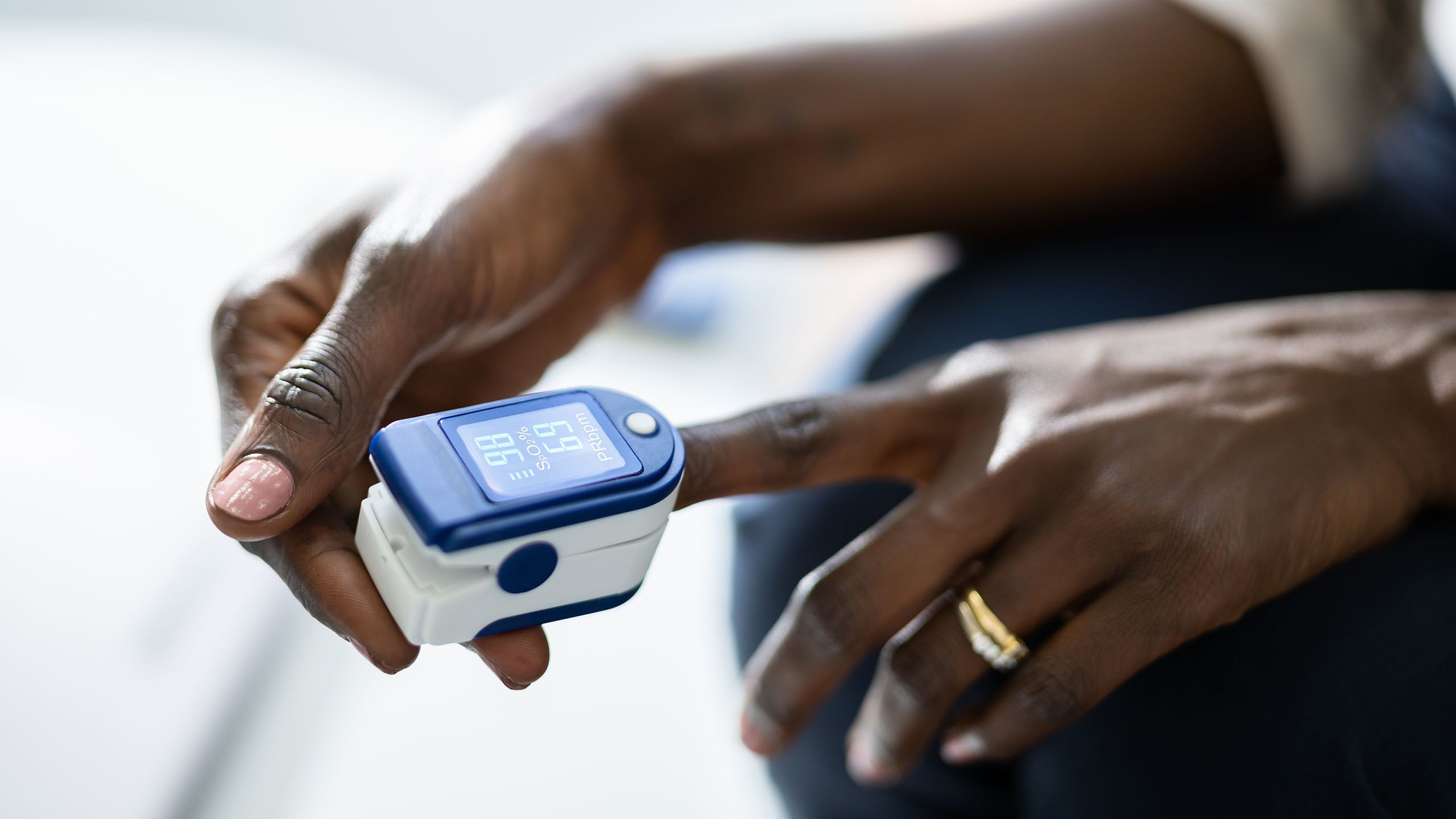
Andrey Popov via iStock/Getty Images
Andrey Popov via iStock/Getty Images
By tackling these biases head-on, the researchers believe they can create better and more inclusive medical devices that not only improve cancer detection and monitoring but also enhance the overall quality and accessibility of healthcare technology.
“Although we’re working on developing and improving technologies, our focus remains the same: we want to improve cancer detection and monitoring, so that every patient is given the best possible chance,” said Else.
Sarah Bohndiek is a Fellow of Corpus Christi College, Cambridge. Thomas Else is a Member of Clare College, Cambridge.
Support cancer research at Cambridge
Cambridge hosts a cancer research community responsible for globally significant breakthroughs and innovations.
Together we are changing the way cancer is treated. Our scientists will make discoveries alongside expert clinicians treating patients, drawing on the world-leading expertise found within the University and across the Cambridge Biomedical Campus.
We will find cancer earlier and treat it better. Join us.
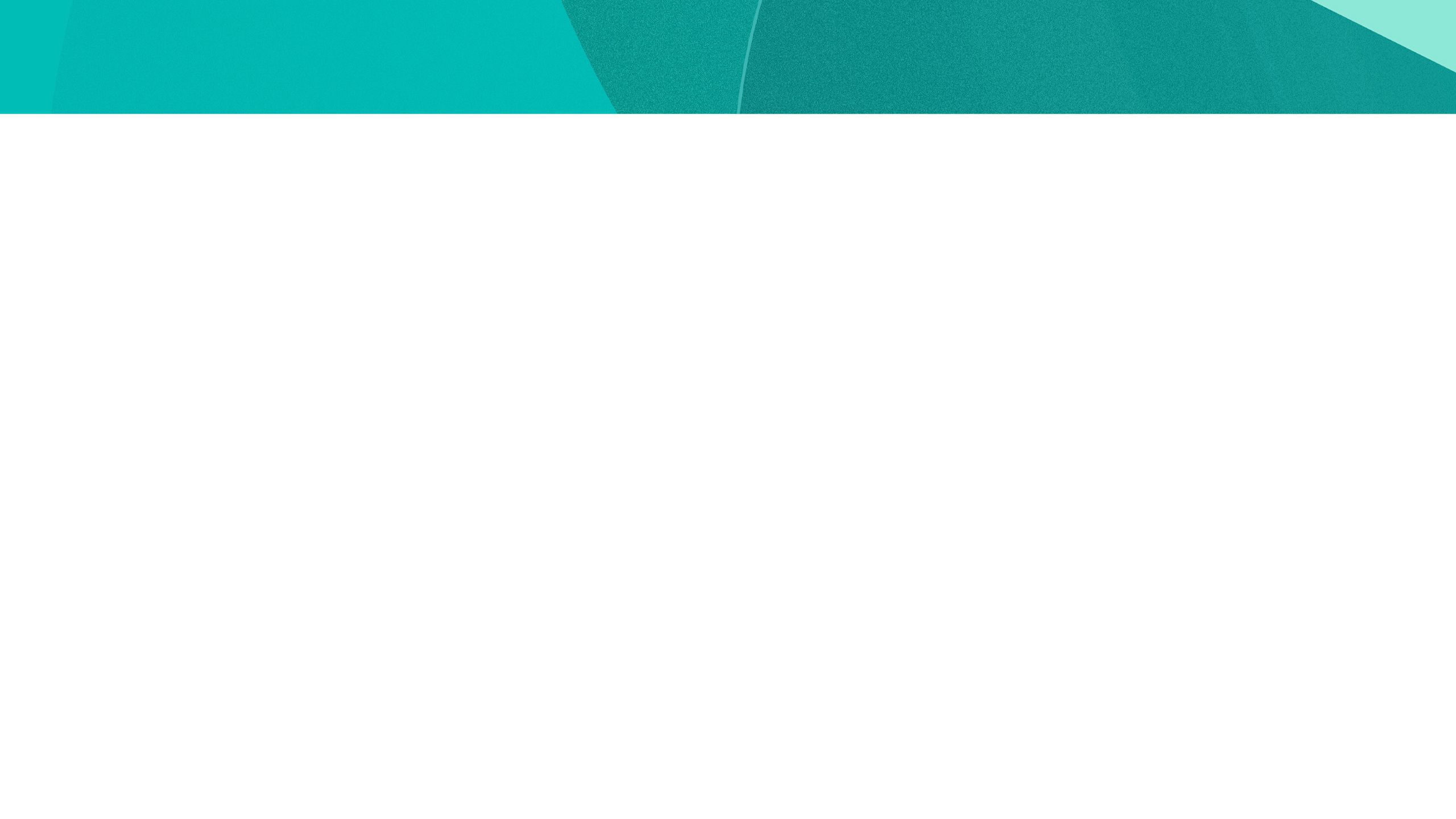
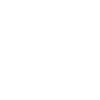