A force to be reckoned with
Gravity is one of the universe's great mysteries. We decided to find out why.
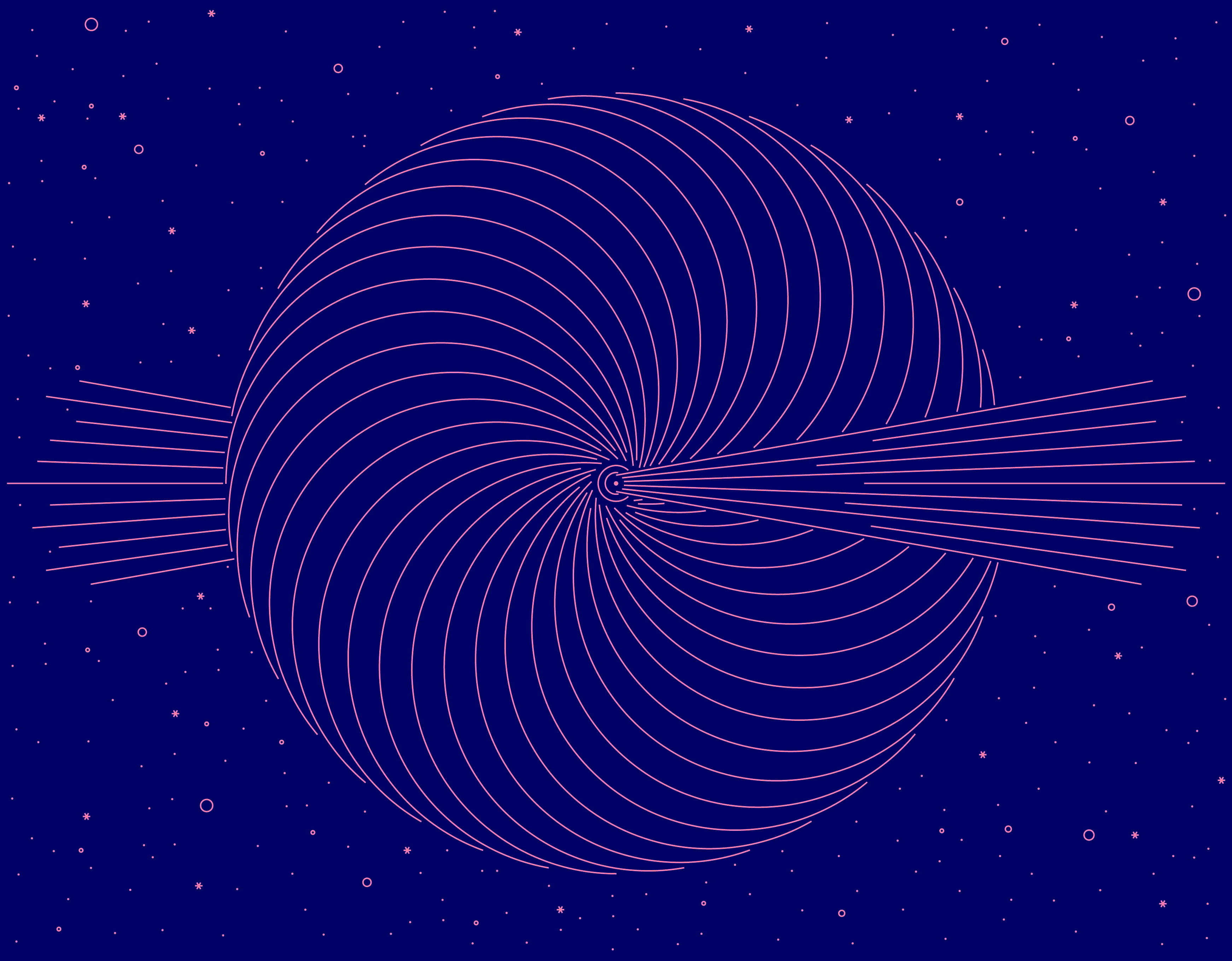
Think you know what gravity is? Think again. New research is revealing how little we know about this most mysterious of forces.
Rumours had been flying round for months. Speculation in tearooms around the world. And at some point towards the end of 2015, Dr Ulrich Sperhake, Lecturer in Theoretical Physics at the Centre for Theoretical Cosmology, began to realise that if this was just a test, he would have heard.Which meant that the rumours must be true. “It was like seeing my football team winning the FA Cup. For the person on the watching shift when it happened, it must have been like winning the cup and Wimbledon wrapped into one!” he says.“Now, I feel more like: ‘Wow. This is a new era in observational physics. Man, how often does that happen?’”
What was the cause of such once-in-a-lifetime excitement? Simply, an extended chirping sound, lasting less than a second, emitting from a monitor in Louisiana, followed by another, identical chirp, heard seven milliseconds later in rural Washington. Twenty milliseconds of data that enabled scientists around the world, including Cambridge researchers working with the Laser Interferometer Gravitational-Wave Observatory, to listen as two mind-blowingly large black holes, 1.2 billion light years away, circled around each other, accelerated from 30 to 250 times a second and then collided,creating one enormous black hole. The impact caused a ripple in space-time – a gravitational wave.
Their findings – the first-ever detection of a ‘binary’black hole, the proof that gravitational waves exist along with a ripple in the space-time continuum that was predicted by Einstein back in 1916 – unlock a new door into our understanding of gravity. And that’s important, because while most of us know about Newton and the apple, gravity remains the great cosmic mystery. Although it was the first force to be described mathematically (by Isaac Newton in 1687), we still do not know how it really works – the best modern description is the general theory of relativity. We know what it does,but not what it is. Which is why Cambridge researchers are working on a number of major projects that aim to find out more about the nature of gravity.
A fundamental force
But to start with, here’s what we do know. First, Newton’s law of gravitation tells us that any two objects in the universe exert a force of attraction on each other. The greater the mass of the two objects and the shorter the distance between them, the stronger the pull of the gravitational forces they exert on each other.
We also know that gravity can work in a complex system with several objects. For example, the Sun exerts gravity on all the planets, and each planet also exerts a force of gravity on the Sun (as well as all the other planets). We know that black holes have so much gravity that light can’t escape their pull, and that their gravitational fields are so powerful that they produce gravitational waves when they collide with each other.
This is why, in the Standard Model of the universe, gravity is described as one of the fundamental forces (alongside electromagnetism, weak nuclear force and strong nuclear force). However, Einstein’s theory of relativity tells us that gravity is more than just a force: it is a curvature in the space-time continuum. In other words, space is not nothing but something, meaning that space must curve around the mass of an object.
An attractive force
But as to what gravity actually is – well that’s where the answers end. Which is why Dr Sperhake is so interested in what happens when black holes collide. “Finding [gravitational] waves means we can observe events that were not clearly identifiable by studying electromagnetism, as it doesn’t exist in a black hole. But gravitational waves can give us that information.
“My work involves looking at the data stream to see if this is all compatible with general relativity and our best guess parameters. To do that we must understand gravitational wave systems in other theories of gravity.”
Richard McMahon, Professor of Astronomy, is focused on determining if and how gravity deviates at the far edges of the universe. His team scans the skies for gravitationally-lensed quasars – quasars whose light is amplified and distorted by matter (such as a cluster of galaxies) between the quasar and the observer.
“The mystery of gravity is that it is an attractive force –it’s actually very weak compared to the other three main forces, but while the others can be both positive and negative and cancel each other out, gravity is attractive, and therefore cumulative, with no way to cancel it out. By discovering quasars and measuring relative distances of the background sources,we can learn if the gravitational force changes.”
Quasars
NASA defines a quasar as 'a very bright object in space that is similar to a star'. Similar, but not the same. Quasars give off more energy than a hundred galaxies, are a trillion times brighter than the Sun, and larger than most solar systems. Sadly, though, they are still not quite bright enough to be seen with the naked eye from Earth.
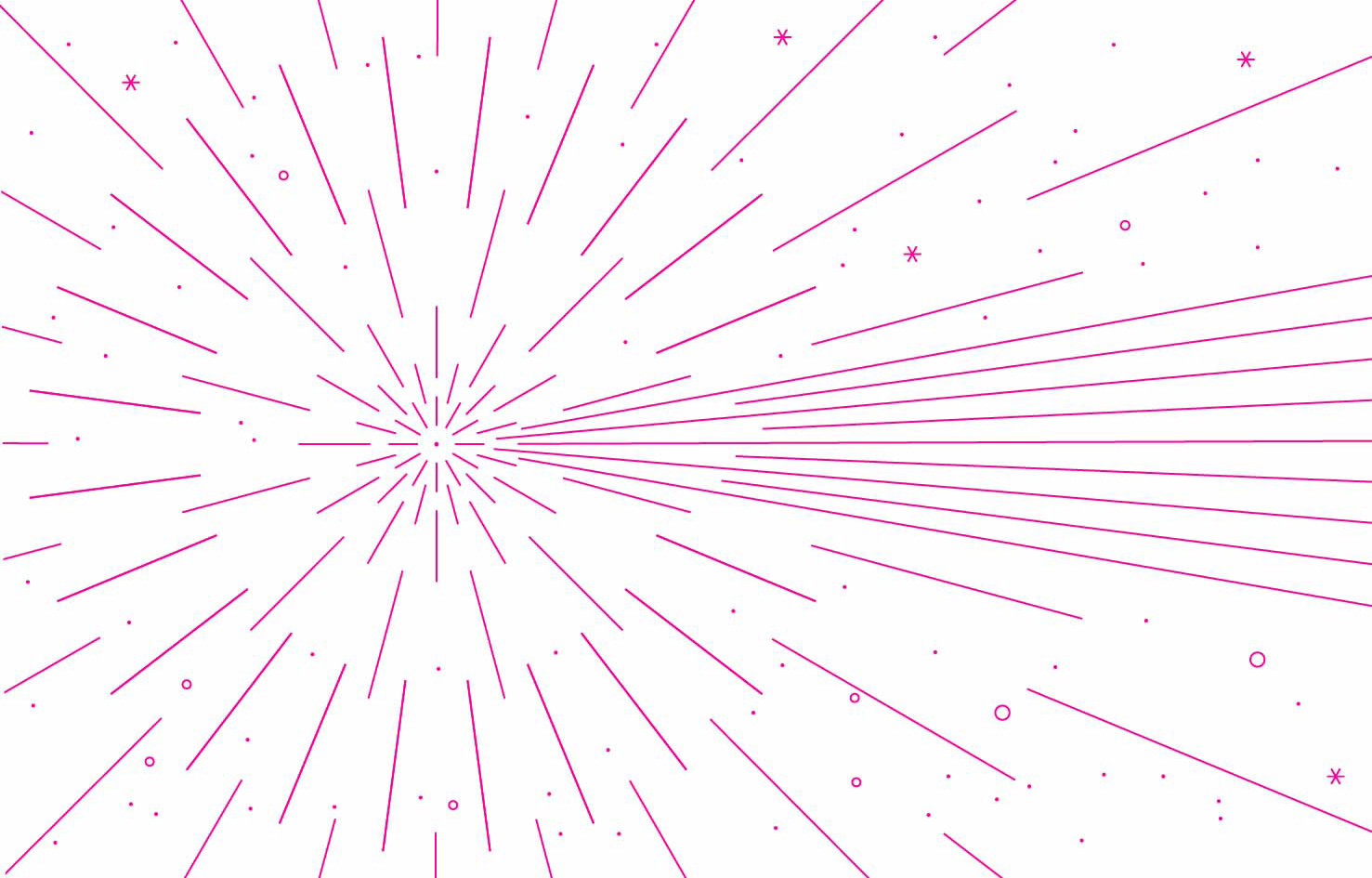
Answers in quasars
Professor McMahon, one of the team of scientists who made the unexpected discovery, in 1998, that the expansion of the universe was accelerating, says the current challenge is about trying to measure gravitational force over a huge distance. “Newtonian gravity has been measured on small scales, such as that of the solar system. But the discovery that the universe is expanding at a greater rate means the Newtonian model is violated at large distances. That could mean that gravity there is weaker or that this accelerated expansion is caused by extra energy – a dark energy we haven’t yet confirmed or identified. And if this is the case, where does this dark energy come from?”
The answers may be found in quasars. “We look at deep optical and infrared images of the sky,” says Professor McMahon. “We measure distance by looking at multiple images of the brightness of these quasars to see if it’s constant, and [then] measure the length of time [light] takes to get to Earth – we’re measuring delayed time five billion light years away.”
Only a handful of quasars have been found so far, but as more and more are discovered, more data becomes available. “We need to find around 100 quasars to really start to build up a picture,” he says. “But that will happen in the next five to 10 years. We’ll monitor them every day – and that will really open up our understanding.”
Dark matter
Dark matter is a hypothetical type of matter, which does not emit or interact with electromagnetic radiation, such as light, and is therefore invisible to the entire electromagnetic spectrum. Although dark matter has not been directly observed, its existence and properties are inferred from its gravitational effects, such as the motions of visible matter, gravitational lensing, its influence on the universe's large-scale structure, on galaxies, and its effects on the cosmic microwave background.
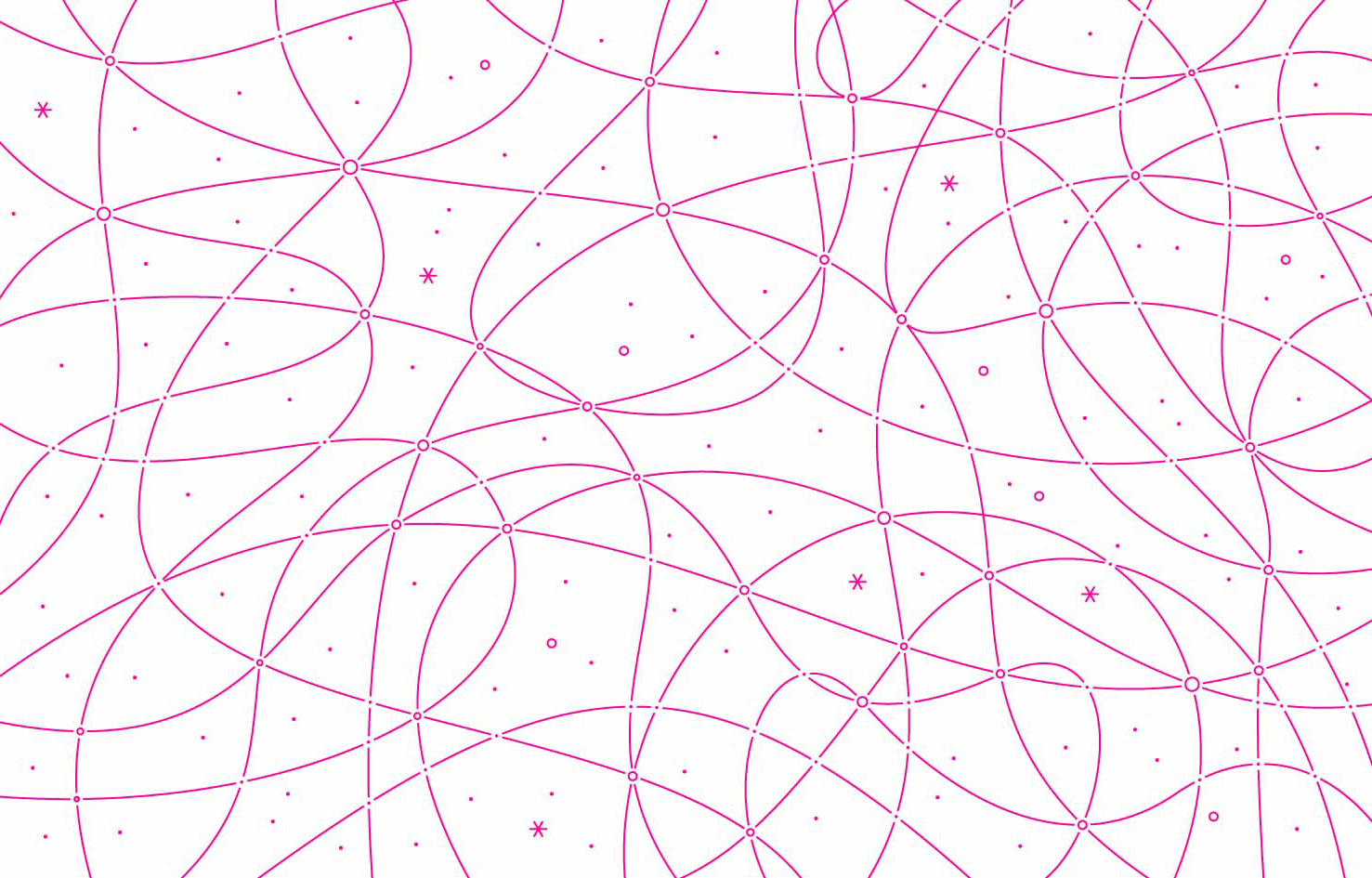
Dark energy, dark matter and baryons
Dr Debora Sijacki, Reader in Astrophysics and Cosmology, is interested in the formation and evolution of cosmic structures, including dark energy, dark matter and baryons (a subatomic particle comprising three quarks).Her theoretical modelling has contributed to the international Illustris Project, which merged a state-of-the-art numerical code with a comprehensive physical model to produce the largest, most accurate, highest definition cosmological simulation thus far of how galaxies were formed.
“One of the goals is to understand the nature of dark matter and dark energy. The more we understand the physics, the closer we are to understanding gravity and what dark energy and dark matter really is,” she says. “In that respect, Illustris is a real breakthrough. Our previous models gave us an idea of how galaxies came about, but their properties did not match reality. Now, thanks to Illustris, we can see the full evolutionary picture of the universe and the emergence of a realistic galaxy population.”
Dr Sijacki adds: “It’s like tuning in a TV – we have a little bit of a fuzzy picture, but it’s a picture that will get clearer. I’m creating novel models which can follow self-consistently the formation and growth of cosmic structures, including dark energy, dark matter and baryons. It is very challenging to model theoretically, and the full complexity of these processes still needs to be unravelled, but ultimately it will allow us to understand the cosmogony of our universe.”
Black hole winds
Black holes feed off material - mostly gas and dust - contained within the 'accretion disk' which surrounds them. But when they eat too much, the accretion disk shoots out hot streams of gas. These streams are black hole winds, reaching temperatures of millions of degrees, travelling at a quarter of the speed of light, with enough power to shut down star formation.
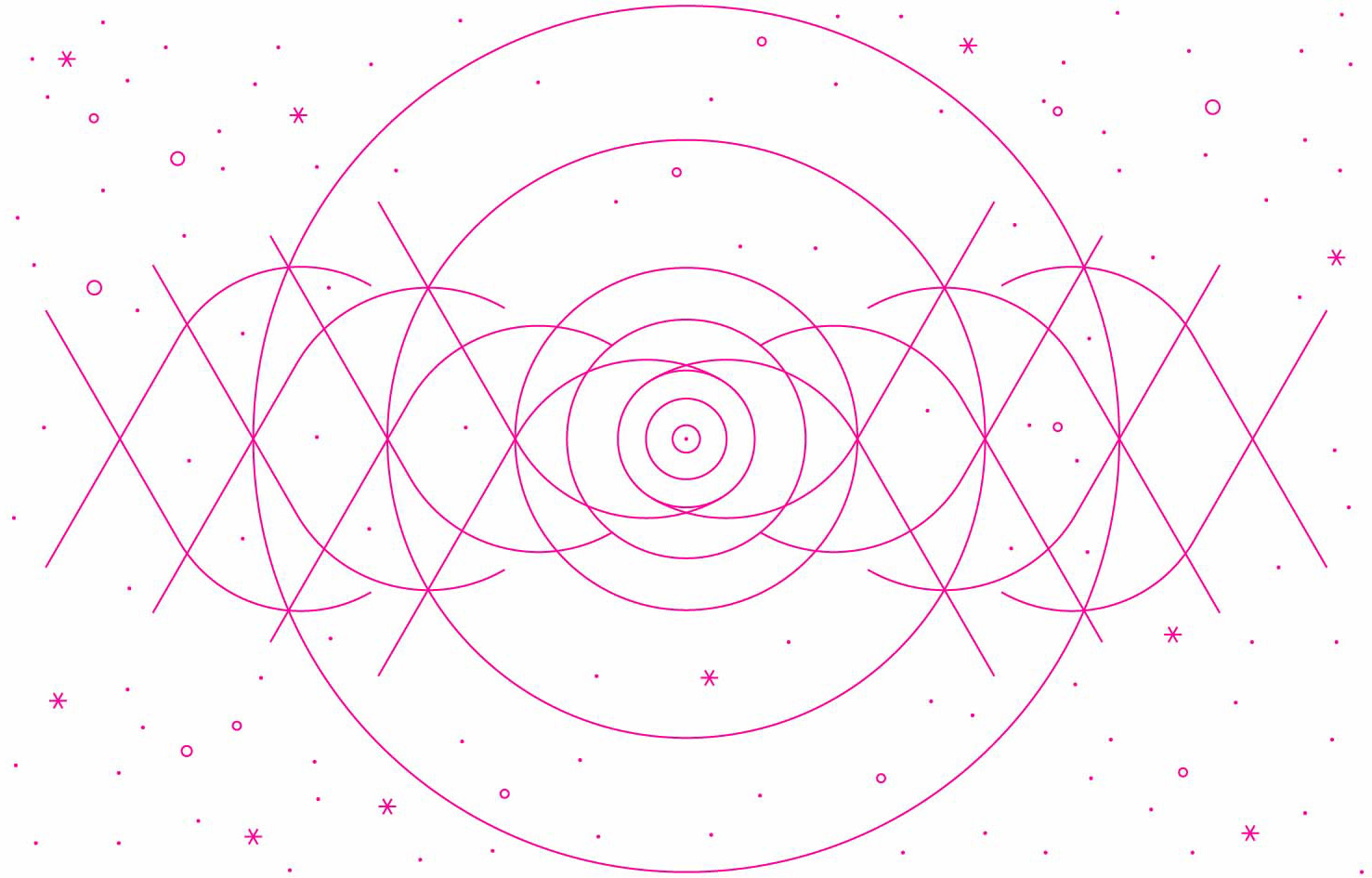
Black holes
Professor Andy Fabian agrees that black holes may hold the key to understanding gravity. His team at the Institute of Astronomy combines theoretical modelling with access to major space-based observatories –including the European Space Agency’s XMM-Newton and NASA’s Chandra and NuSTAR telescopes – to investigate some of the highest energy processes powered by gravity. His research group have made significant recent discoveries. “Black holes are the most extreme of objects, where gravity shuts off a whole part of the universe,” he says. “It’s curious that we see the most intense radiation and luminosity adjacent to something that is completely black. By measuring the mass and spin of black holes we can see how they affect their environment. Energy released by a supermassive black hole at the centre of a galaxy pushes gas out of the galaxy, even though the central black hole is only one billionth the size of the galaxy. It’s like an orange controlling a volume the size of the Earth.
“Last year we took an extended look through the XMM-Newton at a black hole as it was releasing one of the fastest winds we have ever seen.Black holes feed off the surrounding material and sometimes consume too much gas, which is then released as an ultra-fast wind. Matter flows out at a quarter of the speed of light, and we could track how quickly its speed and composition changed with time.
“It’s a eureka moment when something like that happens. You’re all looking at the different sets of data and someone might notice the outflow initially in the iron line, then someone else says: ‘I can see it in the silicon line.’ It wasn’t the first time we’d seen outflow in such an object, but the first time it was observed to vary according to its brightness. This is either because the intense radiation had completely ionized the matter,rendering it transparent, or it could be a geometric effect. But this, together with the observed Doppler shifts, gravitational redshifts, time lags and other signatures of strong gravity in the X-ray data (all of which originate from very close to the black hole), gives us a huge information boost that we’ve never had before. With it, we can better understand how matter flowing into a black hole releases so much energy, and then how some of that matter powers very fast streams of matter. We have so much new information to work with.”
Gravitational waves
In 1916, Albert Einstein came up with the concept of ripples in space-time, or gravitational waves. His theory was that great big things in space - black holes, for example - create these ripples, like a stone thrown into a pond. For almost half a century, scientists were uncertain whether gravitational waves were physically real or just an unphysical coordinate effect. Einstein himself went back and forth between the two viewpoints and it was only after his death that the question was settled in favour of gravitational waves being a genuine physical feature. In September 2015, physicists working on the Laser Interferometer Gravitational-Wave Observatory (LIGO) experiment observed gravitational waves for the first time, created by two black holes colliding.
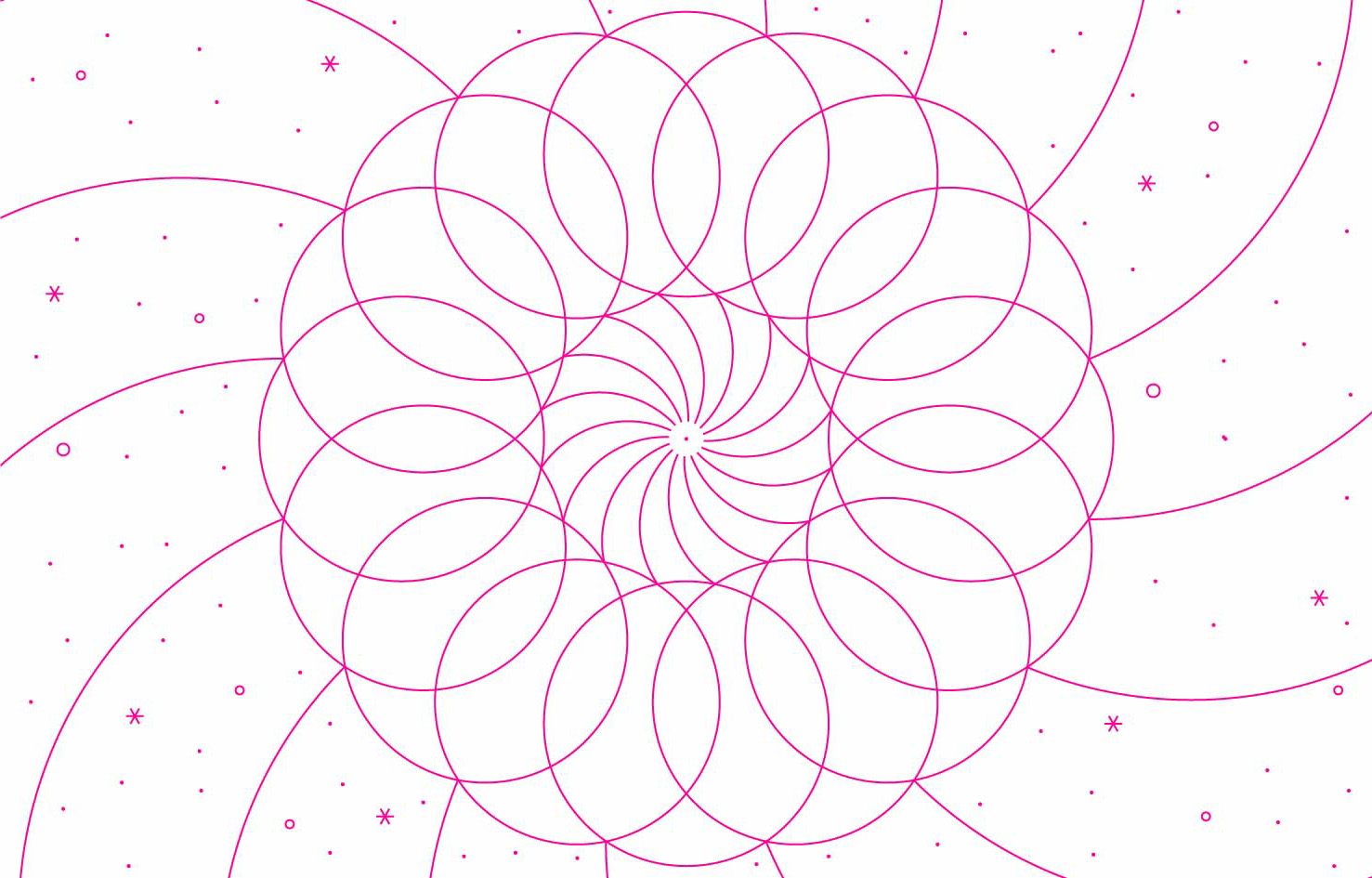
Cosmic microwave background
Anthony Lasenby, Professor of Astrophysics and Cosmology at the Cavendish Laboratory, is observing the cosmic microwave background (CMB) –the residual electromagnetic radiation from the Big Bang, which fills the entire universe – using data generated by Planck, the ESA space-based observatory.
“The data so far revealed by Planck gives us our most precise and quantitative understanding of the cosmos,” Professor Lasenby says. “A main problem in physics is how you reconcile gravity with the fundamental forces of quantum mechanics (electromagnetism and weak and strong nuclear forces). Observation of the CMB helps us to do this by, for example, enabling us to test predictions from modified gravity theories.
“Understanding the amount of dark energy there is is the big focus of the CMB’s contribution to understanding gravity,” adds Professor Lasenby. “The data from Planck can tell you how much dark energy there is, but we still don’t know what it is. The combination of Planck data with other cosmological data to constrain whether the properties of dark energy change with time will be vital in tying down its nature.”
That conflict between gravity and quantum mechanics recently hit the headlines when Dutch Professor Erik Verlinde theorised that gravity isn’t a fundamental force at all, but an emergent phenomenon that is merely a byproduct of other quantum forces – and that gravity around black holes attributed to dark matter is actually an effect of dark energy.
The theory would cast serious doubt on Einstein’s theory of relativity – although it has as many detractors as supporters.
Seismic breakthrough
Nonetheless, many scientists believe recent discoveries will bring us some answers and a greater understanding of gravity – most likely within the next five to 10 years.
“Gravitational waves and quasars will bring so much data,and we’ll have so much more sensitive equipment, that I’ll eat my hat if we don’t get a major surprise soon,” says Professor McMahon. “Even in the worst case, we’ll have so much more data to confirm what we already know.”
Professor Fabian is not so sure. “Einstein’s theory of general relativity and gravity seems to still fit – which makes it all the more remarkable, 100 years on. It would be wonderful to see something that proves it’s wrong, but all our evidence thus far is that he was correct.”
Dr Sperhake would love to see another seismic breakthrough. “A possibly spectacular milestone would be a smoking gun signal pointing towards the need to modify Einstein’s theory. From a theorist’s point of view,it would be great to see such a signature pointing beyond general relativity –but I don’t think that is so likely.”
And if they do find one? “It still doesn’t necessarily mean Einstein was wrong – like Einstein’s theory didn’t make Newton wrong. The Moon landing was calculated with Newton’s theory,” he points out. “Any new discoveries will simply modify the theory.” And gravity – well, for now, at least, gravity remains a mystery.
Read more about Cavendish Astrophysics, the Institute of Astronomy, the Centre for Theoretical Cosmology and the Department of Applied Mathematics and Theoretical Physics.
Find out more about supporting Cambridge research.
Words: Peter Taylor Whiffen
Illustration: La Tigre
Story design: Sarah Collins
This article first appeared in CAM - the
Cambridge Alumni Magazine, issue 82.